Let's develop a more efficient conversion of the sun's light energy into fuels and electric power! There is a possibility of tweaking the photosynthetic reactions to produce fuels we want such as hydrogen, alcohols or even hydrocarbons, rather than carbohydrates, as the photosynthetic reactions produce.
Genetically engineering real plants.
There is a possibility of altering the photosynthetic reactions of cyanobacteria to produce fuels we want such as hydrogen, alcohols or even hydrocarbons, rather than carbohydrates, as they naturally do.
Bacteria are extremely amenable to trans genesis. Genes can be inserted into bacteria with great precision, making expression far easier to control. As a result the desired genetic products can be grown easily under highly controlled conditions, essentially eliminating the danger of transgene escape. The techniques used to slip genes into bacteria chromosomes are identical to those used in gene therapy.
Currently, viruses are the favored vector. Most gene therapies aim to put a new gene into the target genome. When a virus latches onto a cell that isn't somehow protected from the virus, the virus hijacks all the cell's activities for the sole purpose of making more viruses. Viruses reproduce this way because they aren't really alive and have no moving parts of their own to accomplish reproduction. Part of the virus's attack strategy involves integrating virus DNA into the host genome in order to execute viral genome expression.
Gentling a virus for use as a vector involves deleting most of its genes. These deletions effectively rob the virus of almost all of its own DNA, leaving only a few bits. These remaining pieces are primarily the parts normally used by the virus for getting its DNA into the host. Using DNA manipulation techniques, we can splice a healthy gene sequence into the virus to replace the deleted parts of the viral genome. Also a helper is needed to move the payload from the virus to the recipient cell.
Geneticist have several viruses to chose from as possible delivery vectors. These viruses fall into one of two classes:
- Those that integrate their DNA directly into the host genome
- Those that climb into the cell nucleus to become permanent but separate residents (called episomes)
Oncoretroviruses and lentiviruses transfer their genes into the host genome; when the retrovirus genes are in place, they are replicated right along with all the host DNA.
Retroviruses use RNA instead of DNA to code their genes; these viruses use a process called reverse transcription to convert their RNA into DNA, which is then inserted into a host cell's genome.
Adenoviruses are excellent vectors because they pop their genes into cells regardless of whether cell division is occurring. Adenoviruses have been both promising and problematic. On the one hand, these viruses are really good at getting into the host cells. On the other hand, adenoviruses tend to excite a strong immune response. Adenoviruses do not put their DNA directly into the host genome. Instead, they exist separately as episomes, so they are aren't as likely to cause mutations as lentiviruses. The drawback is that the episomes aren't always replicated and passed on the daughter cells when the host cell divides. Nonetheless, adenovirus vectors have been used with notable success.
Geneticists participating in the project will have to suggest the best choice for delivery vectors after our team will develop the S P E C S for the
G E N E T I C A L Y - E N G I N E R E D - C Y A N O B A C T E R I A
to produce directly alcohol (ethanol) through photosynthesis.
To create the S P E C, the physiology of ethanol producing photosynthesis will have to be drafted, proteins including.
With the protein draft in hand, geneticists will work backwards from the building blocks of that protein, to specify the amino acids, to discern what the mRNA instructions has to be.
Mr.Takeo Miki suggested:
Current energy production from biomass has a low efficiency due to energy losses at various levels in cellular metabolism. However, this process has the attractive feature of automatic self-reproduction, so that for the long term energy budget it is not necessary to invest energy in renewing the photosynthetic apparatus.
To engineer from scratch a self-reproducing biological solar energy converter with maximized efficiency, a combination of systems-biology- and synthetic-biology approaches will be required. This should be a long-term concerted effort in which geneticists, physiologists, molecular biologists, biochemists, physicists, and computational experts should join forces to maximize the translation of atomic-level understanding to high yield solar to fuel conversion and coupling it with downstream production processes.
This will require understanding of the living cell as a self-regulating, self-ordering, self-maintaining and adaptive entity and will eventually lead to a merger of life science and materials science. Life is based on interplay between diversification at the bottom, through random mutations, and selection from the top (i.e. at the organism level). Currently the basic understanding is still lacking regarding how to implement a smart matrix that provides the appropriate selection criteria for development, (genetic) adaptation and evolution into artificial systems with the desired properties. Bacteria generally live in ecosystems where tasks often are distributed over a multitude of species, and the steady state is maintained on a multi-organism level with high metabolic energy cost, in part because of the fluctuating environmental conditions. Alternatively, bacteria have become organelles like chloroplasts, embedded in a host matrix (eukaryotic plant cell) with lower metabolic costs.
Several cyanobacterial genomes have been sequenced already at an early stage in the genomics revolution, since 1996, and the investigation of their systems biology properties is progressing well. Chlamydomonas reinhardtii, a recently sequenced single cell green alga, has been put forward as the “cell factory of the future” and biotechnology has produced variants that accumulate high quantities of polysaccharides. Time has come to design an “artificial organism” at the drawing table, including the minimum of pathways that are required to produce an on-board solar energy to fuel system to drive cell-factories in an efficient way.
Cell factories with an on-board light-energy conversion system with optimal efficiency and convenience, can produce a wide variety of chemicals and chemical feedstock’s in a sustainable manner. In this way efficiency gain and solar energy utilization go hand in hand.
Minimally metabolizing units will provide optimal production of desired target compounds including biomass; this includes systems in which biological waste can be converted to nutritious materials with self-reproducing devices containing only a single photo system. To develop this, a major challenge is to provide comprehensive insight into the systems biology of relevant photosynthetic organisms to provide a sound basis for the new design of photosynthetic cell factories. Major hurdles will be to understand and implement:
- Minimally metabolizing smart matrices
- Proper (genetic) adaptation modules, for guiding development and evolution towards the desired function.
As an alternative, the design of an artificial chloroplast-type entity (i.e. without self-reproduction capability) embedded in a host matrix could be considered. This may further improve the efficiency.
The overall success of this approach will also depend on the inclusion of methods that allow for an efficient harvesting of end products, such as spontaneous aggregation of products, self sedimentation at the end of the (artificial) life cycle and facile drying of regimented biomass.
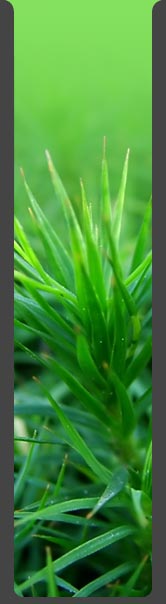
Comments
Your Turn
Mr Michael Malagiero, in fact, proposes a new track for us. His suggestion follows:
Anaerobic photosynthetic bacteria have the capacity to produce significant amounts of H2 by using sunlight. This H2 can be produced either by hydrogenising or nitrogenising. The bacteria use reductant derived either from solar powered reactions or the oxidation of organic compounds to drive H2 production. By using sunlight and waste organic materials - for example sewage, agricultural or animal wastes - as electron donors to support the growth of bacteria and their subsequent generation of H2, these procedures will not only produce solar-powered H2 but have the added environmental benefit of treating and removing waste products in a carbon neutral process.....(Please see more under Enzymes) Michael Malagiero, Oct 31
To engineer from scratch a self-reproducing biological solar energy converter with maximized efficiency, a combination of systems-biology- and synthetic-biology approaches will be required. This should be a long-term concerted effort in which geneticists, physiologists, molecular biologists, biochemists, physicists, and computational experts should join forces to maximize the translation of atomic-level understanding to high yield solar to fuel conversion and coupling it with downstream production processes. Takeo Miki, Oct 26
Thanks to
Ethanol-bio-fuel.com Andrea Toth, Oct 14th